Additional Resources
General
Version published: 
November 28, 2023 - 10:16am
- Pacific Northwest Blue Carbon Working Group
- Coastal Carbon Research Coordination Network
- International Partnership for Blue Carbon
- The Blue Carbon Initiative
- The Blue Carbon National Working Group
- Blue Carbon for Our Planet Act
- The Blue Boat Initiative
- Blue Carbon Network (Pew)
- Blue Carbon Lab (Deakin University)
- Blue Carbon Canada (UN Decade Action)
- Global Mangrove Watch
- Blue Carbon Calculator
- Blue Carbon Explorer (TNC) (Map)
- Coastal Carbon Atlas
- Blue Carbon Action Partnership
- Global Ocean Decade Programme for Blue Carbon (UN Decade Program)
- Blue Ventures (GEM etc)
- Blue Carbon Accelerator Fund
- Sea4soCiety
- Pacific Northwest Blue Carbon Working Group
- Coastal Carbon Research Coordination Network
- International Partnership for Blue Carbon
- The Blue Carbon Initiative
- The Blue Carbon National Working Group
- Blue Carbon for Our Planet Act
- The Blue Boat Initiative
- Blue Carbon Network (Pew)
- Blue Carbon Lab (Deakin University)
- Blue Carbon Canada (UN Decade Action)
- Global Mangrove Watch
- Blue Carbon Calculator
- Blue Carbon Explorer (TNC) (Map)
- Coastal Carbon Atlas
- Blue Carbon Action Partnership
- Global Ocean Decade Programme for Blue Carbon (UN Decade Program)
- Blue Ventures (GEM etc)
- Blue Carbon Accelerator Fund
- Sea4soCiety
- Pacific Northwest Blue Carbon Working Group
- Coastal Carbon Research Coordination Network
- International Partnership for Blue Carbon
- The Blue Carbon Initiative
- The Blue Carbon National Working Group
- Blue Carbon for Our Planet Act
- The Blue Boat Initiative
- Blue Carbon Network (Pew)
- Blue Carbon Lab (Deakin University)
- Blue Carbon Canada (UN Decade Action)
- Global Mangrove Watch
- Blue Carbon Calculator
- Blue Carbon Explorer (TNC) (Map)
- Coastal Carbon Atlas
- Blue Carbon Action Partnership
- Global Ocean Decade Programme for Blue Carbon (UN Decade Program)
- Blue Ventures (GEM etc)
- Blue Carbon Accelerator Fund
- Sea4soCiety
- Pacific Northwest Blue Carbon Working Group
- Coastal Carbon Research Coordination Network
- International Partnership for Blue Carbon
- The Blue Carbon Initiative
- The Blue Carbon National Working Group
- Blue Carbon for Our Planet Act
- The Blue Boat Initiative
- Blue Carbon Network (Pew)
- Blue Carbon Lab (Deakin University)
- Blue Carbon Canada (UN Decade Action)
- Global Mangrove Watch
- Blue Carbon Calculator
- Blue Carbon Explorer (TNC) (Map)
- Coastal Carbon Atlas
- Blue Carbon Action Partnership
- Global Ocean Decade Programme for Blue Carbon (UN Decade Program)
- Blue Ventures (GEM etc)
- Blue Carbon Accelerator Fund
- Sea4soCiety
No projects listed. Want to add a project to this section? Become a Contributor.
Examples of Mangrove Forest Restoration Efforts
Version published: 
November 28, 2023 - 10:18am
- Delta Blue Carbon (Pakistan)
- Global Mangrove Alliance
- Mangrove Action Project (US)
- Blue Carbon Lab (Australia)
- Vida Manglar Carbon Project (Colombia)
- Delta Blue Carbon (Pakistan)
- Global Mangrove Alliance
- Mangrove Action Project (US)
- Blue Carbon Lab (Australia)
- Vida Manglar Carbon Project (Colombia)
- Delta Blue Carbon (Pakistan)
- Global Mangrove Alliance
- Mangrove Action Project (US)
- Blue Carbon Lab (Australia)
- Delta Blue Carbon (Pakistan)
- Global Mangrove Alliance
- Mangrove Action Project (US)
- Blue Carbon Lab (Australia)
- Vida Manglar Carbon Project (Colombia)
- Delta Blue Carbon (Pakistan)
- Global Mangrove Alliance
- Mangrove Action Project (US)
- Blue Carbon Lab (Australia)
- Vida Manglar Carbon Project (Colombia)
- Delta Blue Carbon (Pakistan)
- Global Mangrove Alliance
- Mangrove Action Project (US)
- Blue Carbon Lab (Australia)
- Vida Manglar Carbon Project (Colombia)
- Delta Blue Carbon (Pakistan)
- Global Mangrove Alliance
- Mangrove Action Project (US)
- Blue Carbon Lab (Australia)
- Vida Manglar Carbon Project (Colombia)
Microalgae cultivation for carbon dioxide removal (CDR) is emerging as a potential solution to the climate crisis. Microalgae are fast-growing organisms that convert carbon dioxide (CO2) into biomass and various other organic compounds through photosynthesis. Microalgae play a critical role in the global carbon cycle, capable of fixing CO2 10 - 50 times more efficiently than terrestrial plants . Proposed strategies to utilize microalgae for carbon dioxide removal take advantage of the physiology of microalgae and their role in the carbon cycle and seek to achieve long term (>100 years) sequestration and storage of carbon. The two primary strategies for microalgae CDR are:
- Open systems where the open ocean is directly manipulated to enhance biological production, capture atmospheric CO2, and export the captured carbon to the deep ocean. In open systems, CO2 fixation is facilitated by the addition of limiting macronutrients (e.g., phosphorus, nitrogen, silica) and/or micronutrients (e.g., iron) to the ocean’s surface to augment biological production . Open system techniques accelerate natural processes already occurring in the ocean. Most approaches in the open ocean fall into the following two categories (some proposals that do not fit into these categories are also explored).
- Surface nutrient addition: the direct addition of nutrients (macro or micro) into ocean waters in situ to increase microalgal growth
- Nutrient upwelling: artificial upwelling of nutrient-rich deep ocean waters to the surface to increase microalgal growth
- Closed systems where inputs and growth conditions are controlled, and outputs (microalgae) are harvested within the confines of a pond or a photobioreactor. In closed systems, CO2 fixation is facilitated by the mixing of required inputs (sunlight, nutrients, CO2, water) and the introduction of microalgae culture with the intention of reproduction and continuous fixation in a contained system . This can be accomplished on shore in cultivation ponds or photobioreactors, or afloat in photobioreactors either stationary or towed at sea .
-
- Onshore: encompasses more established methods of microalgae cultivation, including photobioreactors, cultivation ponds, and hybrid onshore configurations. In these systems, all inputs are tightly controlled and regulated, and outputs must be directly managed through either storage or utilization of byproducts. While cultivation techniques are well-established and show high technological readiness, storage and utilization pathways remain underdeveloped and scale is a major consideration. Social and environmental risks for closed onshore systems are easier to monitor and mitigate due to the controlled nature of the system.
- Offshore: includes floating photobioreactors (PBRs) that are incorporated into a floating platform which can be stationary or towed behind a ship. In these systems, cultivation occurs in the photobioreactor, inputs are regulated, and outputs can be actively managed or directed. While at sea, these photobioreactors operate much like their onshore counterparts to cultivate microalgae, however nutrients and energy are provided by the ocean water and wave action, respectively. After microalgae are cultivated, they can be sunk into the deep ocean for sequestration or hauled to shore to be used as biomass. This is an area that has garnered much attention in startup communities (see this Y Combinator request for startups), however, little is available about the technologies in the open-sourced or peer reviewed literature.
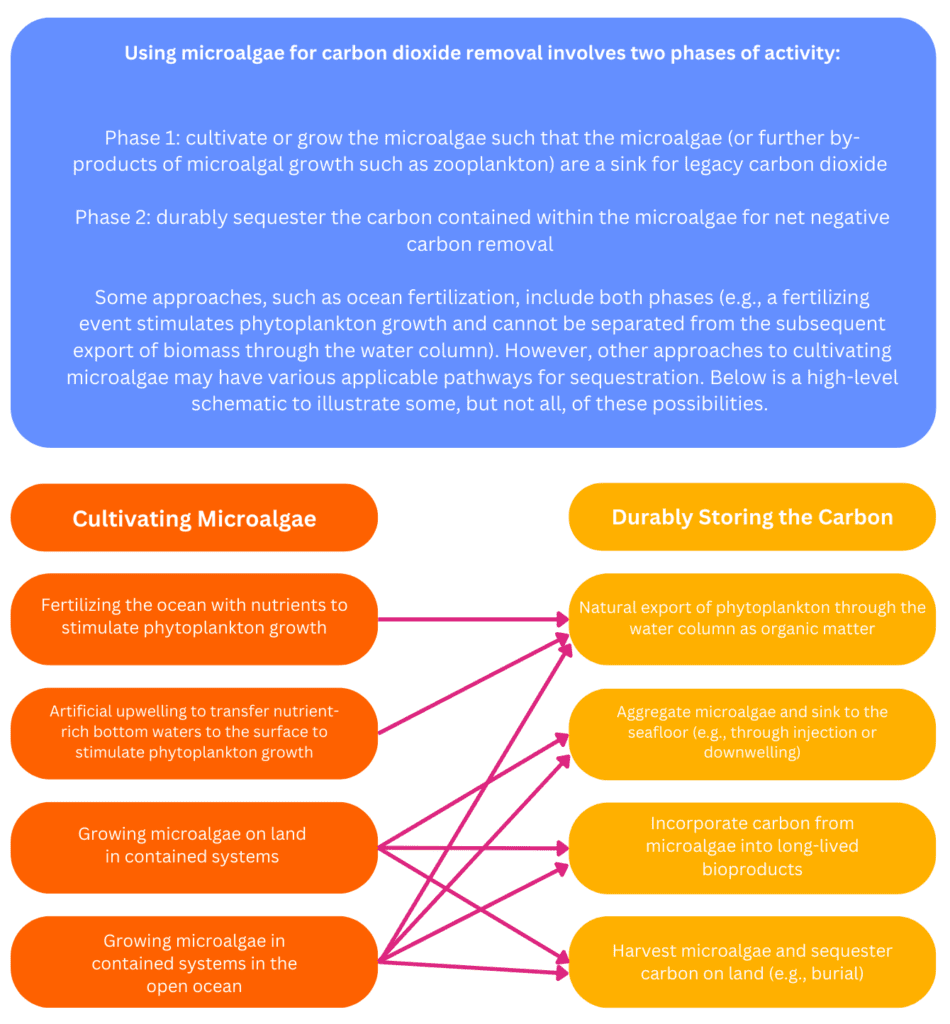
Microalgae cultivation for carbon dioxide removal (CDR) is emerging as a potential solution to the climate crisis. Microalgae are fast-growing organisms that convert carbon dioxide (CO2) into biomass and various other organic compounds through photosynthesis. Microalgae play a critical role in the global carbon cycle, capable of fixing CO2 10 - 50 times more efficiently than terrestrial plants . Proposed strategies to utilize microalgae for carbon dioxide removal take advantage of the physiology of microalgae and their role in the carbon cycle and seek to achieve long term (>100 years) sequestration and storage of carbon. The two primary strategies for microalgae CDR are:
- Open systems where the open ocean is directly manipulated to enhance biological production, capture atmospheric CO2, and export the captured carbon to the deep ocean. In open systems, CO2 fixation is facilitated by the addition of limiting macronutrients (e.g., phosphorus, nitrogen, silica) and/or micronutrients (e.g., iron) to the ocean’s surface to augment biological production . Open system techniques accelerate natural processes already occurring in the ocean. Most approaches in the open ocean fall into the following two categories (some proposals that do not fit into these categories are also explored).
- Surface nutrient addition: the direct addition of nutrients (macro or micro) into ocean waters in situ to increase microalgal growth
- Nutrient upwelling: artificial upwelling of nutrient-rich deep ocean waters to the surface to increase microalgal growth
- Closed systems where inputs and growth conditions are controlled, and outputs (microalgae) are harvested within the confines of a pond or a photobioreactor. In closed systems, CO2 fixation is facilitated by the mixing of required inputs (sunlight, nutrients, CO2, water) and the introduction of microalgae culture with the intention of reproduction and continuous fixation in a contained system . This can be accomplished on shore in cultivation ponds or photobioreactors, or afloat in photobioreactors either stationary or towed at sea .
-
- Onshore: encompasses more established methods of microalgae cultivation, including photobioreactors, cultivation ponds, and hybrid onshore configurations. In these systems, all inputs are tightly controlled and regulated, and outputs must be directly managed through either storage or utilization of byproducts. While cultivation techniques are well-established and show high technological readiness, storage and utilization pathways remain underdeveloped and scale is a major consideration. Social and environmental risks for closed onshore systems are easier to monitor and mitigate due to the controlled nature of the system.
- Offshore: includes floating photobioreactors (PBRs) that are incorporated into a floating platform which can be stationary or towed behind a ship. In these systems, cultivation occurs in the photobioreactor, inputs are regulated, and outputs can be actively managed or directed. While at sea, these photobioreactors operate much like their onshore counterparts to cultivate microalgae, however nutrients and energy are provided by the ocean water and wave action, respectively. After microalgae are cultivated, they can be sunk into the deep ocean for sequestration or hauled to shore to be used as biomass. This is an area that has garnered much attention in startup communities (see this Y Combinator request for startups), however, little is available about the technologies in the open-sourced or peer reviewed literature.
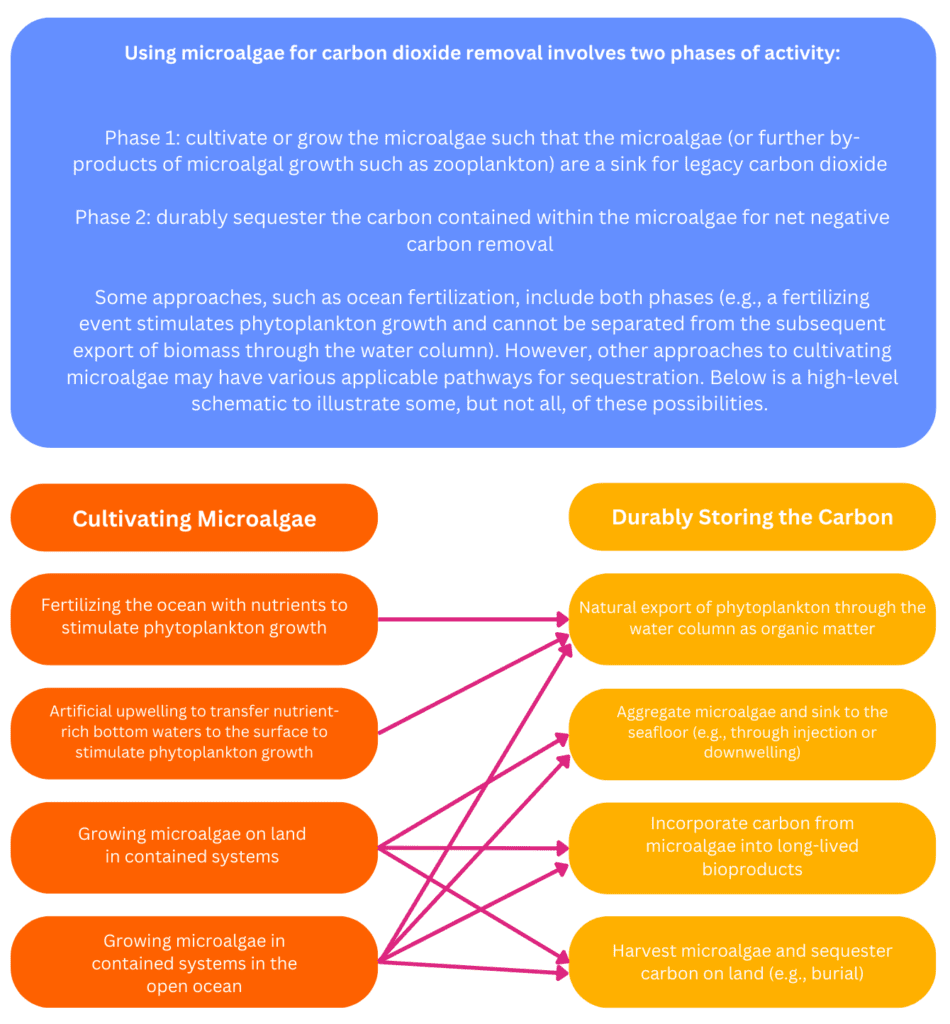
No projects listed. Want to add a project to this section? Become a Contributor.
Examples of Seagrass Restoration Efforts
Version published: 
November 28, 2023 - 10:19am
- Ocean Alive (Portugal)
- MedGardens (Mallorca)
- ResilienSEA (West Africa)
- Ozfish (Australia)
- Project Seagrass (UK & Globally)
- For an example of a successful seagrass bed restoration, see Orth et al. 2020[1]https://www.science.org/doi/10.1126/sciadv.abc6434 .
- Ocean Alive (Portugal)
- MedGardens (Mallorca)
- ResilienSEA (West Africa)
- Ozfish (Australia)
- Project Seagrass (UK & Globally)
- For an example of a successful seagrass bed restoration, see Orth et al. 2020[1]https://www.science.org/doi/10.1126/sciadv.abc6434 .
- Ocean Alive (Portugal)
- MedGardens (Mallorca)
- ResilienSEA (West Africa)
- Ozfish (Australia)
- Project Seagrass (UK & Globally)
- For an example of a successful seagrass bed restoration, see Orth et al. 2020.
- Ocean Alive (Portugal)
- MedGardens (Mallorca)
- ResilienSEA (West Africa)
- Ozfish (Australia)
- Project Seagrass (UK & Globally)
- For an example of a successful seagrass bed restoration, see Orth et al. 2020[1]https://www.science.org/doi/10.1126/sciadv.abc6434 .
- Ocean Alive (Portugal)
- MedGardens (Mallorca)
- ResilienSEA (West Africa)
- Ozfish (Australia)
- Project Seagrass (UK & Globally)
- For an example of a successful seagrass bed restoration, see Orth et al. 2020[1]https://www.science.org/doi/10.1126/sciadv.abc6434 .
- Ocean Alive (Portugal)
- MedGardens (Mallorca)
- ResilienSEA (West Africa)
- Ozfish (Australia)
- Project Seagrass (UK & Globally)
- For an example of a successful seagrass bed restoration, see Orth et al. 2020.
- Ocean Alive (Portugal)
- MedGardens (Mallorca)
- ResilienSEA (West Africa)
- Ozfish (Australia)
- Project Seagrass (UK & Globally)
- For an example of a successful seagrass bed restoration, see Orth et al. 2020[1]https://www.science.org/doi/10.1126/sciadv.abc6434 .
No projects listed. Want to add a project to this section? Become a Contributor.
Examples of Salt Marsh Restoration Efforts
Version published: 
November 28, 2023 - 11:33pm
Microalgae cultivation for carbon dioxide removal (CDR) is emerging as a potential solution to the climate crisis. Microalgae are fast-growing organisms that convert carbon dioxide (CO2) into biomass and various other organic compounds through photosynthesis. Microalgae play a critical role in the global carbon cycle, capable of fixing CO2 10 - 50 times more efficiently than terrestrial plants . Proposed strategies to utilize microalgae for carbon dioxide removal take advantage of the physiology of microalgae and their role in the carbon cycle and seek to achieve long term (>100 years) sequestration and storage of carbon. The two primary strategies for microalgae CDR are:
- Open systems where the open ocean is directly manipulated to enhance biological production, capture atmospheric CO2, and export the captured carbon to the deep ocean. In open systems, CO2 fixation is facilitated by the addition of limiting macronutrients (e.g., phosphorus, nitrogen, silica) and/or micronutrients (e.g., iron) to the ocean’s surface to augment biological production . Open system techniques accelerate natural processes already occurring in the ocean. Most approaches in the open ocean fall into the following two categories (some proposals that do not fit into these categories are also explored).
- Surface nutrient addition: the direct addition of nutrients (macro or micro) into ocean waters in situ to increase microalgal growth
- Nutrient upwelling: artificial upwelling of nutrient-rich deep ocean waters to the surface to increase microalgal growth
- Closed systems where inputs and growth conditions are controlled, and outputs (microalgae) are harvested within the confines of a pond or a photobioreactor. In closed systems, CO2 fixation is facilitated by the mixing of required inputs (sunlight, nutrients, CO2, water) and the introduction of microalgae culture with the intention of reproduction and continuous fixation in a contained system . This can be accomplished on shore in cultivation ponds or photobioreactors, or afloat in photobioreactors either stationary or towed at sea .
-
- Onshore: encompasses more established methods of microalgae cultivation, including photobioreactors, cultivation ponds, and hybrid onshore configurations. In these systems, all inputs are tightly controlled and regulated, and outputs must be directly managed through either storage or utilization of byproducts. While cultivation techniques are well-established and show high technological readiness, storage and utilization pathways remain underdeveloped and scale is a major consideration. Social and environmental risks for closed onshore systems are easier to monitor and mitigate due to the controlled nature of the system.
- Offshore: includes floating photobioreactors (PBRs) that are incorporated into a floating platform which can be stationary or towed behind a ship. In these systems, cultivation occurs in the photobioreactor, inputs are regulated, and outputs can be actively managed or directed. While at sea, these photobioreactors operate much like their onshore counterparts to cultivate microalgae, however nutrients and energy are provided by the ocean water and wave action, respectively. After microalgae are cultivated, they can be sunk into the deep ocean for sequestration or hauled to shore to be used as biomass. This is an area that has garnered much attention in startup communities (see this Y Combinator request for startups), however, little is available about the technologies in the open-sourced or peer reviewed literature.
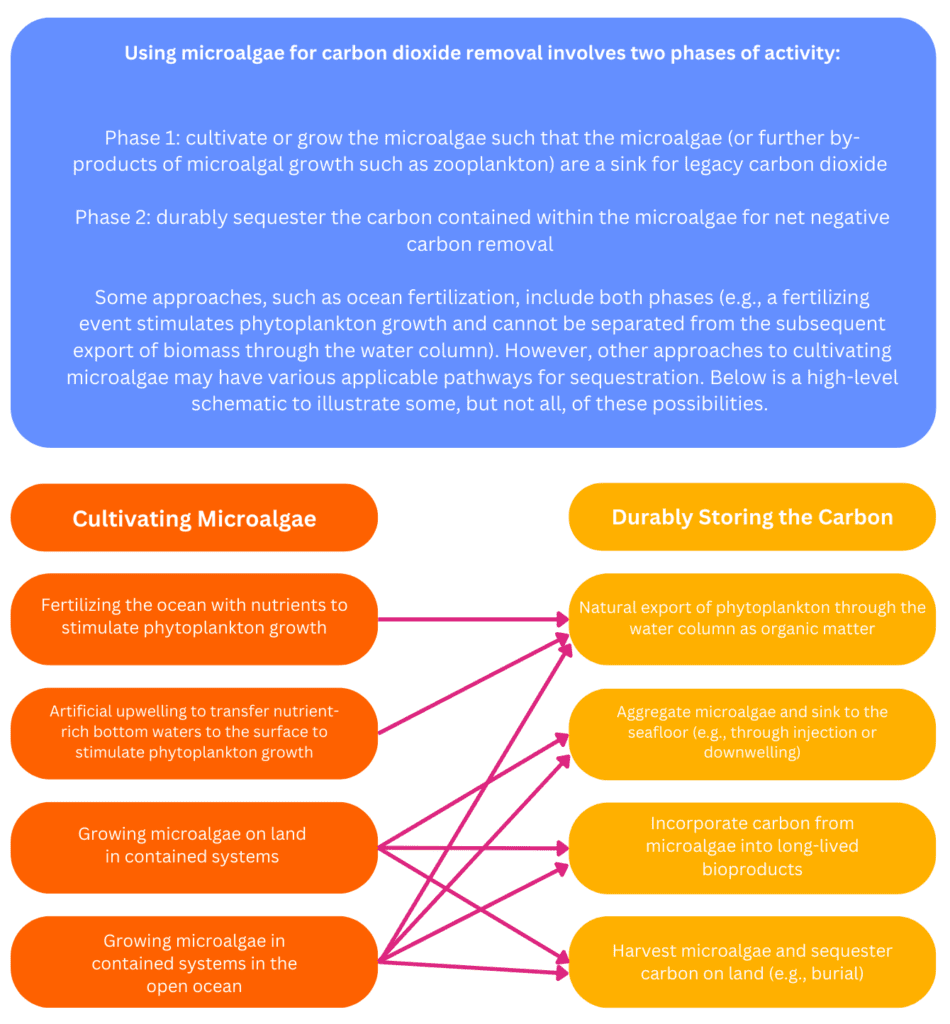
Microalgae cultivation for carbon dioxide removal (CDR) is emerging as a potential solution to the climate crisis. Microalgae are fast-growing organisms that convert carbon dioxide (CO2) into biomass and various other organic compounds through photosynthesis. Microalgae play a critical role in the global carbon cycle, capable of fixing CO2 10 - 50 times more efficiently than terrestrial plants . Proposed strategies to utilize microalgae for carbon dioxide removal take advantage of the physiology of microalgae and their role in the carbon cycle and seek to achieve long term (>100 years) sequestration and storage of carbon. The two primary strategies for microalgae CDR are:
- Open systems where the open ocean is directly manipulated to enhance biological production, capture atmospheric CO2, and export the captured carbon to the deep ocean. In open systems, CO2 fixation is facilitated by the addition of limiting macronutrients (e.g., phosphorus, nitrogen, silica) and/or micronutrients (e.g., iron) to the ocean’s surface to augment biological production . Open system techniques accelerate natural processes already occurring in the ocean. Most approaches in the open ocean fall into the following two categories (some proposals that do not fit into these categories are also explored).
- Surface nutrient addition: the direct addition of nutrients (macro or micro) into ocean waters in situ to increase microalgal growth
- Nutrient upwelling: artificial upwelling of nutrient-rich deep ocean waters to the surface to increase microalgal growth
- Closed systems where inputs and growth conditions are controlled, and outputs (microalgae) are harvested within the confines of a pond or a photobioreactor. In closed systems, CO2 fixation is facilitated by the mixing of required inputs (sunlight, nutrients, CO2, water) and the introduction of microalgae culture with the intention of reproduction and continuous fixation in a contained system . This can be accomplished on shore in cultivation ponds or photobioreactors, or afloat in photobioreactors either stationary or towed at sea .
-
- Onshore: encompasses more established methods of microalgae cultivation, including photobioreactors, cultivation ponds, and hybrid onshore configurations. In these systems, all inputs are tightly controlled and regulated, and outputs must be directly managed through either storage or utilization of byproducts. While cultivation techniques are well-established and show high technological readiness, storage and utilization pathways remain underdeveloped and scale is a major consideration. Social and environmental risks for closed onshore systems are easier to monitor and mitigate due to the controlled nature of the system.
- Offshore: includes floating photobioreactors (PBRs) that are incorporated into a floating platform which can be stationary or towed behind a ship. In these systems, cultivation occurs in the photobioreactor, inputs are regulated, and outputs can be actively managed or directed. While at sea, these photobioreactors operate much like their onshore counterparts to cultivate microalgae, however nutrients and energy are provided by the ocean water and wave action, respectively. After microalgae are cultivated, they can be sunk into the deep ocean for sequestration or hauled to shore to be used as biomass. This is an area that has garnered much attention in startup communities (see this Y Combinator request for startups), however, little is available about the technologies in the open-sourced or peer reviewed literature.
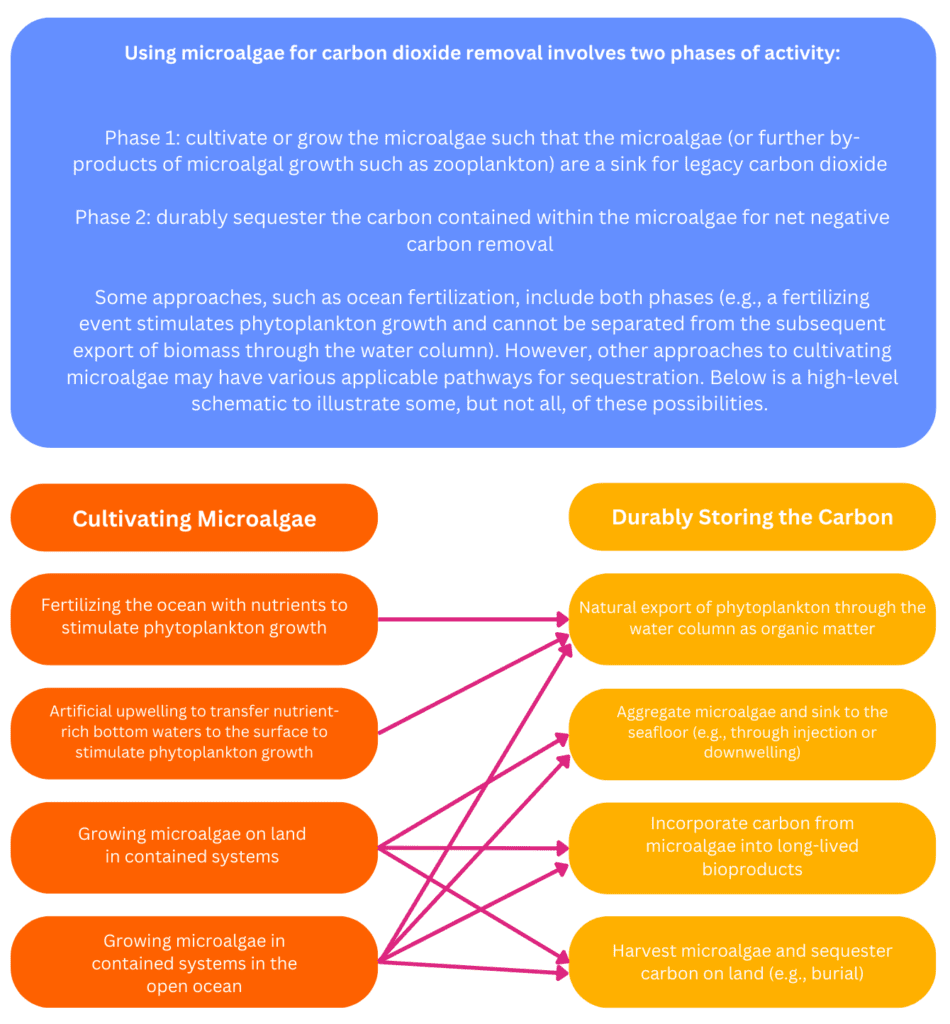
No projects listed. Want to add a project to this section? Become a Contributor.
Help advance Ocean-based CDR road maps. Submit Comments or Content
How to submit comments:
Thanks for helping to advance these roadmaps. Here is how it works!
Or, send general feedback to info@oceanvisions.org
All submitted comments will be reviewed by the Ocean Visions team to ensure that they are true, verifiable, and additive before being added to the road maps. You can increase the likelihood that your comments will be incorporated into the road maps by providing references (where appropriate).