The term “blue carbon” has historically referred to three (vascularly) vegetated coastal ecosystems: mangrove forests, salt marshes, and seagrass beds{{1}}. These ecosystems are among the most productive in the world{{2}} and sequester carbon in their underlying substrates (e.g., soil or sediment). Estimates of their global distribution reach ~36-185 million hectares, storing as much as 33 billion tons of carbon{{3}}, however there remain large knowledge gaps around spatial extent and storage capacity, owing to the high variability across regions and ecosystems. The carbon in the substrate can be stored as organic or inorganic carbon and can remain sequestered for millennia{{4}},{{5}}, positioning these ecosystems as important parts of the global carbon cycle. Situated between land and water, these ecosystems also play critical roles in human society and provide many services including coastal buffering from storms, surges and erosion control generally, nursery grounds for fish, culturally significant sites, and sources of income from fishing and tourism. Blue carbon ecosystems have gained attention as levers for climate change mitigation, including carbon sequestration{{6}}.
Some of this attention is related to the major uptick in interest and attention around carbon dioxide removal (CDR){{7}}, which has again raised questions about the capacity of blue carbon ecosystems to sequester carbon and combat climate change{{8}},{{9}},{{10}},{{11}}. This discussion has also broadened the conversation around the definition of blue carbon, with the IPCC (2019){{12}} defining blue carbon as ‘all biologically driven carbon fluxes and storage in marine systems that are amenable to management’. This definition can and has been interpreted to encompass not only coastal vegetated ecosystems that store carbon in their structure and sediments, but also other marine life such as fish and mammals, as well as natural seaweed stands and seaweed farms - all systems that not only store and recycle carbon through their living tissues, but also respond to anthropogenic manipulation and management. Two notable examples of this broadened definition include the National Academies of Sciences, Engineering, and Medicine’s (NASEM) 2022 report on ocean carbon dioxide removal in which they include a chapter on “Recovery of Marine Ecosystems” for CDR (including benthic, pelagic, and offshore ocean systems along with mangrove forests, salt marshes, and seagrass beds) and the National Oceanographic and Atmospheric Administration’s (NOAA) 2023 Strategy for Carbon Dioxide Removal Research which includes coastal blue carbon (defined as mangrove forests, salt marshes, and seagrass beds) as well as ‘Marine Ecosystem Recovery’ (fishes and marine mammals). (Also note NOAA, 2021.).
Following these recent advances, this road map will consider carbon stored in natural seaweed stands and in marine animals (e.g., fishes and mammals) in the definition of blue carbon, alongside mangrove forests, salt marshes, and seagrass beds. Natural seaweed stands and marine animals have received substantially less attention as potential pathways for additional carbon dioxide sequestration and will require accelerated research to determine their potential. The nascent nature of these pathways makes them particularly well suited to be assessed in this road map.
Note: While the blue carbon definition above could be interpreted to include microalgae cultivation, we have excluded it from consideration in this road map because we have already released a road map for Microalgae Cultivation and Carbon Sequestration. In addition, we limit discussion of seaweed (macroalgae) farms to coastal farms which passively sequester carbon through biomass export to underlying sediments or offshore to deeper waters. We exclude discussion of seaweed farming coupled to active sequestration (such as sinking or harvesting to produce biochar) because this topic is covered in a different road map, Macroalgae Cultivation and Carbon Sequestration.
This road map focuses on assessing the potential for blue carbon to contribute to carbon dioxide removal (CDR), or the additional sequestration of carbon, and what is needed to achieve that potential. In many cases, this will look like the large-scale restoration of ecosystems or rebuilding of animal populations (and, in some cases, expansion of ecosystems or animal populations beyond past limits). Recent work by the Environmental Defense Fund and the High Level Panel for a Sustainable Ocean Economy suggests that even the most rigorous and optimistic future estimates of blue carbon restoration may yield small gains in additional carbon sequestration when compared with the scale of anthropogenic emissions and other possible CDR pathways{{13}},{{14}},{{15}},{{16}}. One reason for this is the spatial limitation presented by the restoration of coastal vegetated ecosystems (i.e., mangrove forests, seagrass beds, and salt marshes). These ecosystems have spatial limits on their potential for restoration, and thus, their potential for carbon removal, however other sources of blue carbon, such as animal biomass and seaweed farms, are far less limited by spatial constraints. Moreover, blue carbon restoration offers low or no risk pathways, which sets it apart from other proposed CDR solutions. Not only do restoration efforts of blue carbon pose fewer risks to the environment and society than other proposed CDR solutions, but they also offer an enormous capacity for other benefits to both ecosystems and communities. In this way, carbon removal may in fact be best thought of as a “co-benefit” to blue carbon restoration and efforts{{17}}. Regardless, there is still critical research that would increase our understanding of the role blue carbon plays in the carbon cycle and inform responsible decision making at the ocean-climate nexus.
In light of current and future climate disruption and risk, there is a growing need for solutions that will help to remove and durably store legacy greenhouse gas emissions. However, there remain a substantial set of unanswered questions around the capacity and efficacy of carbon storage in blue carbon systems. As scientists, practitioners, and policy makers continue investigating blue carbon as a climate solution, it is critical to be clear about what is known, what remains unknown, and identify a pathway by which to answer the most important outlying questions. This road map lays out the current state of knowledge around carbon sequestration and storage in blue carbon systems, details the most pressing unanswered questions to understand the potential for blue carbon sequestration, and proposes ways in which to accelerate the development and testing of these potential solution pathways.
A Note on Restoration vs. Protection
Protection of existing blue carbon ecosystems and their embedded carbon avoid emissions generated as these systems degrade. This is complementary and distinct from restoration of blue carbon systems, which is regrowth of these ecosystems. This regrowth is where additional carbon can be sequestered. Because the focus of this road map is the potential for blue carbon systems to contribute to carbon dioxide removal, this map will only focus on restoration efforts, their state of progress, challenges, knowledge gaps, and opportunities to drive forward progress.
A Note on Units of Measurement
This road map differs from the other Ocean Visions’ Ocean CDR Road Maps in its use of ‘Gigaton CO2 equivalent’ (Gt CO2e) in order to account for the important role played by non-CO2 greenhouse gases (e.g., methane, CH4, and nitrous oxide, N2O) within blue carbon systems. Although petagrams (Pg) is a unit commonly used in blue carbon literature, we use the equivalent gigatons (or gigatonnes; Gt) in this road map.
The Environmental Defense Fund published three detailed reports in 2022 on "Natural Climate Solutions" that examine the roles that coastal blue carbon, natural seaweed stands, and marine animals may play in the overall climate solutions landscape, including, but not limited to, carbon removal. For more in-depth reading about any one of these systems and the other roles they can play beyond carbon removal, we recommend exploring the recent suite of detailed reports (linked below) as well as the many cited papers throughout this road map. There are also several other resources embedded in this road map including links to blue carbon working groups around the globe where current research and work is happening and interactive maps showing known extents of blue carbon habitats.
|
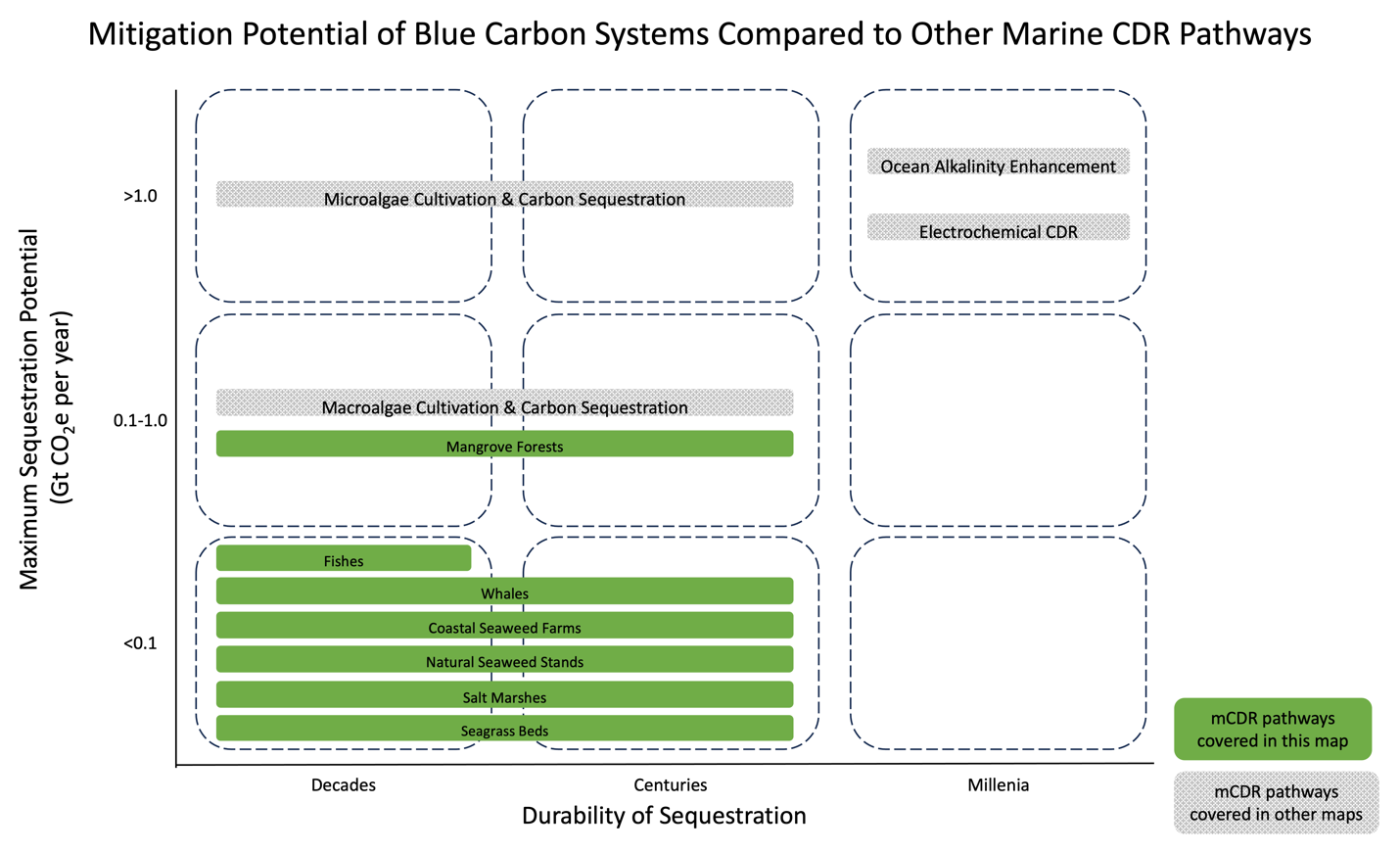
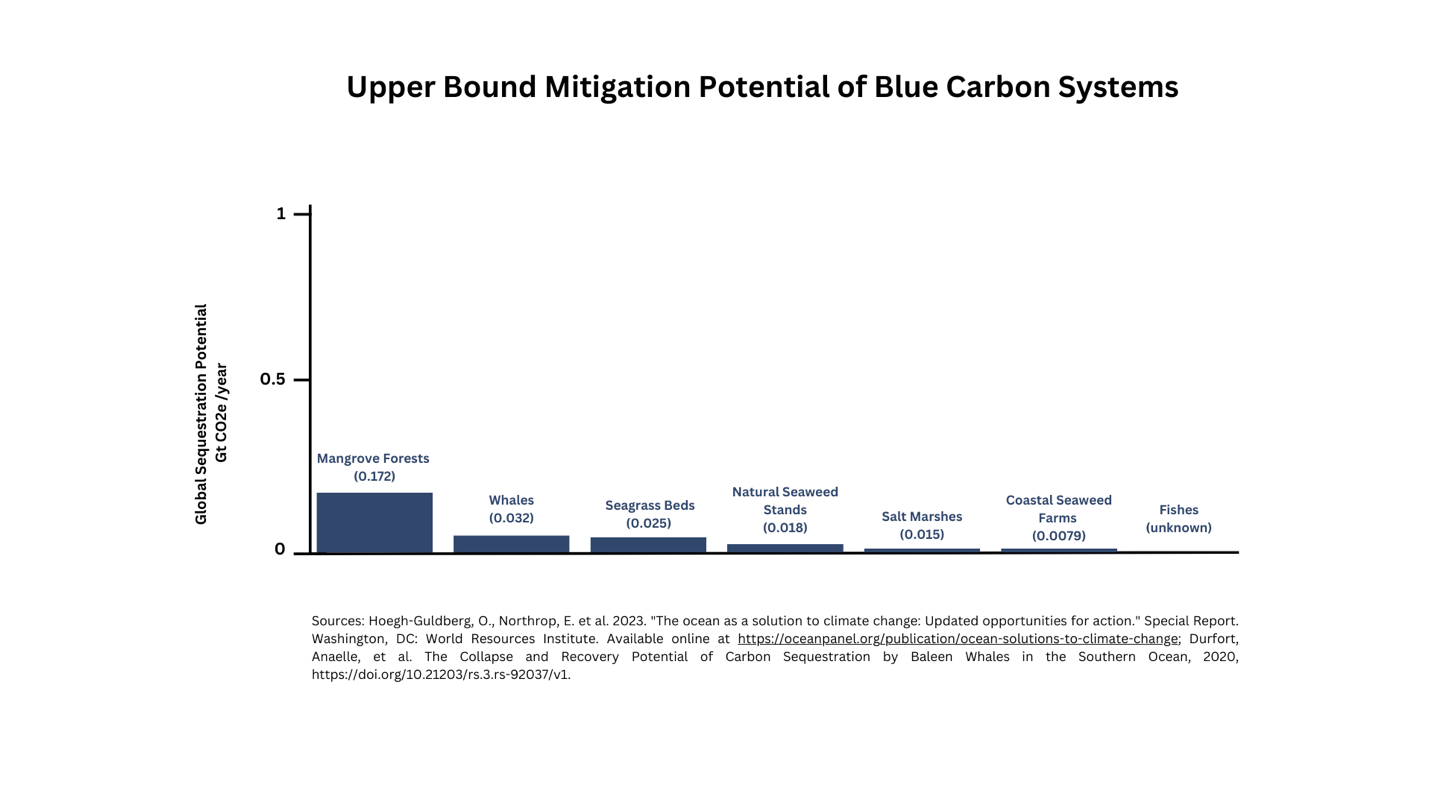
Environmental Co-Benefits
|
|||||||||||
Co-Benefit |
System |
||||||||||
Animal Carbon |
Natural Seaweed Stands |
Mangrove Forests |
Salt Marshes |
Seagrass Beds |
|||||||
Amelioration of ocean acidification |
(Mongin, 2016) |
(Banjeree & Paul, 2022) |
(Ricart et al., 2022; Koweek et al. 2018) |
||||||||
Potential for ocean alkalinity enhancement |
(Fakhraee et al., 2023) |
(Fakhraee et al., 2023) |
|||||||||
Contribution to biodiversity |
(Theuerkauf et al., 2021) |
(Thrush et al., 2013; Esteves 2014) |
(McHenry et al. 2021) |
||||||||
Contribution to habitat provisioning |
(Langton et al., 2019) |
||||||||||
Contribution to heavy metal and nutrient pollution removal |
(Zheng et al, 2019; Jiang et al., 2020) |
(Lee et al. 2019) |
|||||||||
Contribution to fishery enhancement |
(Rimmer et al., 2021; Theuerkauf et al., 2021) |
(Orth et al., 2006) |
|||||||||
Improved water clarity through the facilitation of settlement of fine sediments |
(Jiang et al., 2020) |
||||||||||
Coastal protection against erosion and/or sea level rise |
(Elsmore et al., 2023) |
(Barbier et al., 2011) |
(Barbier et al., 2011) |
(Barbier et al., 2011, Duarte et al. 2013) |
|||||||
Nutrient cycling |
(Le Mézo et al. 2022; Ratnarajah et al., 2014)
|
(Cotas et al., 2023)
|
(Mitra 2019) |
(Sousa et al., 2010) |
(Orth et al., 2006) |
||||||
Reducing biodiversity loss |
(NASEM 2022 mCDR) |
(NASEM 2022 mCDR) |
(NASEM 2022 mCDR) |
(NASEM 2022 mCDR) |
|||||||
Restoring the role of marine organisms in the carbon cycle |
(NASEM 2022 mCDR) |
(NASEM 2022 mCDR) |
(NASEM 2022 mCDR) |
(NASEM 2022 mCDR) |
(NASEM 2022 mCDR) |
Mangrove forests, salt marshes, and seagrass beds grow where land meets the sea and store carbon in their vegetation and underlying sediments/soils. The sediments/soils can store both organic and inorganic carbon. Some of this carbon originates from within the ecosystem itself (autochthonous carbon) while some originates from outside the ecosystem (allochthonous carbon). The exact proportion of autochthonous versus allochthonous carbon is difficult to determine, and differs by site, but is important when trying to account for all the carbon fluxes in a system. What’s more, carbon can move laterally and vertically in, out, and through these systems, creating additional complexity for carbon accounting.
Of these three ecosystems, in terms of current global extent, current carbon storage, and carbon sequestration potential, mangrove forests are the best understood followed by salt marshes. Seagrass beds are the least well understood both in spatial extent and ultimate carbon sequestration potential.
Restoration and its limitations: CDR pathways within coastal vegetated ecosystems are primarily concerned with restoration – the act of bringing an ecosystem back as nearly as possible to its original condition{{1}}. However, the goal of restoration may be unattainable for several reasons, including irreversible land and hydrological (starved of freshwater input) change, conditions that do not permit rehabilitation, and the inherent spatial limitations for ecosystems that occupy specific niches{{2}}. Another challenge is the host of known and unknown consequences of climate change which may impact the feasibility and efficacy of restoration efforts. Monitoring of restoration projects will also be critical both as important data points for studies and predictive science as well as for any involvement with carbon markets. There has been historical reluctance from both government agencies and private developers to fund long-term monitoring{{3}}, however this will be crucial for informed decision making about where and how to implement restoration projects.
Net sequestration of CO2e and its storage durability are key metrics when considering restoration projects for CDR. Ideally, monitoring of restoration projects should feed back into adaptive management practices, where management techniques are adjusted though an iterative process in response to other variables such as climate change.
[post_title] => Overview [post_excerpt] => [post_status] => publish [comment_status] => closed [ping_status] => closed [post_password] => [post_name] => overview [to_ping] => [pinged] => [post_modified] => 2023-12-01 18:18:01 [post_modified_gmt] => 2023-12-01 18:18:01 [post_content_filtered] => [post_parent] => 4033 [guid] => http://ocean-visions.local/roadmaps/living-blue-carbon/introduction-to-the-roadmap/overview-2/ [menu_order] => 0 [post_type] => page [post_mime_type] => [comment_count] => 0 [filter] => raw )Mechanism for CDR
- Restoring hydrology: Restoration of hydrology, through reversing drainage or removing tidal restrictions may assist in the recovery and expansion of existing mangrove sites. This path may be met with more resistance (especially political and social) than other types of restoration efforts.
- Planting mangrove seedlings: This is one of the most common restoration techniques, however, large-scale planting efforts have often failed{{1}}, (many of these shortcomings a result of projects that do not adequately consider environmental and socioeconomic conditions{{2}}) and smaller projects may not deliver carbon storage on climate-relevant scales{{3}}.
-
- Research is being done on the efficacy of automated mangrove planting via drones{{4}},{{5}}. This work is still in early stages but may provide lower cost options for planting efforts, especially in difficult to access locations.
- Restoration efforts should be de-risked in smaller projects before moving to larger projects. This will require investment in capacity-building in communities and institutions, and mechanisms to match restoration opportunities with prospective supporters and investors{{6}}.
CDR Potential
Estimated Sequestration Potential: 0.028 – 0.172 Gt CO2e/year{{7}}
Sequestration Durability: Decades -- 1,000 years{{8}} (Durability of sequestered carbon will depend on multiple variables and can vary greatly between individual ecosystems)
Challenges
- Accounting:
-
- Estimating future sequestration potentials or future rates of carbon sequestration is more difficult than estimating their current global extent and storage.
- While there is general scientific consensus that mangroves are net sinks of CO2{{9}}, there is growing research that indicates production of CH4 and N2O nonuniformly across habitats. In order to have accurate calculations of greenhouse gas budgets, these emissions will need to be included in the accounting{{10}}.
- Current estimates of sequestration potential do not account for the effects of climate change on the ecosystem (e.g., warming, sea level rise, coastal squeeze, increased tropical storms).
- Mangroves are inextricably connected to other marine ecosystems like seagrasses and coral reefs, complicating the definition of boundaries for estimating “additional” carbon sequestration and introducing other complications, such as figuring out how much carbon is autochthonous and how much is allochthonous. Additionally, many carbon markets currently require that all carbon be autochthonous to count toward credits or have poorly defined frameworks for whether to credit for allochthonous carbon that accumulates on site as a result of restoration or management actions.
- Restoration:
-
- There are high costs associated with restoration efforts{{11}}.
- Mangrove ecosystems are heterogeneous across geographies, and this must be accounted for when assessing individual habitat potential for carbon sequestration. (e.g., findings from one location/ecosystem may not be directly applicable to other location/ecosystems
- There is work being done to use unmanned aerial systems (UAS) to assess mangrove estuaries on the Pacific Coast of Costa Rica{{12}} and in Malaysia{{13}}, enabling monitoring of difficult to access locations. This type of innovation may be important for monitoring and verification of restored mangrove forests.
Key Knowledge Gaps
- Quantitative understanding of the impacts of climate change on mangrove ecosystems and how these may affect restoration efforts.
- Incomplete understanding of greenhouse gas production and release (e.g., CH4 and N2O) by and from mangroves and how this impacts net carbon removal.
- Uncertainty around durability of carbon stored in mangroves and their sediments as well as carbon burial rates{{14}}.
First-Order Priorities
*Adapted from the Environmental Defense Fund report on Coastal Natural Climate Solutions{{15}}
- Improve predictions of the effects of climate change on mangrove forests*
- Predictions needed include:
- Effects of increased tropical storm intensity and frequency on the destruction and degradation of mangrove ecosystems
- The timescales over which any previously sequestered carbon could be released to the atmosphere
- Understand/map the potential spatial extent of mangrove forests given climate change impacts on existing habitats and poleward migration of mangroves with future warming.
- Predictions needed include:
- Improved, spatially explicit modeling approaches to predict greenhouse gas fluxes and net carbon sequestration*
- Models should function across different mangrove ecosystems whose sediment dynamics cannot be resolved solely using imagery taken from space or aircraft.
- The development of carbon crediting projects requires we know not only how much carbon a mangrove ecosystem contains in its above and belowground biomass, but also the magnitude of various greenhouse gas fluxes from underlying soils or sediments at present and in the future.
- These models should account for future ecosystem state due to the interactive effects of climate change and predicted land-use changes, including the phenomenon of coastal squeeze, the prevention of expansion due to rural areas on one side and the ocean on the other.
- Create studies designed to better differentiate between carbon originating from within the system (autochthonous) and carbon originating from elsewhere (allochthonous)*
- The ability to distinguish among carbon sources is particularly critical since methodologies developed under the voluntary carbon market standards require deduction of allochthonous carbon from any claimed credit.
- Accelerate the development of technology and biotechnology that can help scale restoration efforts
- Optimize drones and autonomous robots for increased scalability of mangrove planting.
- Test biological engineering techniques to enhance carbon storage within mangrove forests.
Mechanism for CDR
- Most restoration efforts include recovery of tidal exchange (e.g., restoring hydrology and tidal flow), managed realignment, and sediment level amendment{{1}}. Efforts can also include removal of non-native vegetation and/or replanting of native vegetation.
- For an in-depth look at salt marsh restoration efforts to date, refer to Adam 2019 “Salt Marsh Restoration”{{2}}
CDR Potential
Estimated Sequestration Potential: 0.004 – 0.015 Gt CO2e/year{{3}}
Sequestration Durability: Decades -- 1,000 years{{4}} (Durability of sequestered carbon will depend on multiple variables and can vary greatly between individual ecosystems)
Challenges
- Little potential for expansion of current habitats due to extensive land conversion.
- Gaining access to suitable land for restoration projects may be difficult and expensive.
- Determining baseline historical extent for setting restoration objectives.
Key Knowledge Gaps
- Need accurate predictions of carbon sequestration which requires integrated modeling of ecological impacts, land-use, and climate effects (made more challenging by high variability in carbon burial rates{{5}}).
- Discerning between competing feedbacks associated with climate and sea level rise, which can have both positive and negative impacts on ecosystems.
- Measurement of greenhouse gases other than CO2 (e.g., CH4 and N2O) that are produced and their significance to net sequestration.
- What is the fate of carbon produced outside of marshes and trapped in them?
- Total global areal existing extent remains poorly mapped, especially at high latitudes.
First-Order Priorities
*Adapted from the Environmental Defense Fund report on Coastal Natural Climate Solutions{{6}}
- Conduct research to better understand and characterize the multiple carbon fluxes within salt marsh systems
- Improve predictions of carbon sequestered by salt marshes through modeling*
- Better knowledge of the relative contributions of carbon produced outside of (allochthonous) and within (autochthonous) marshes to salt marsh organic carbon pools, and the labilities of the carbon derived from these two sources*
- Investigate and characterize climate impacts, both positive and negative, on salt marsh systems*
- Sea level rise, warming, and increased coastal storm frequency and severity all need further investigation.
- Measure non-CO2 greenhouse gases that are produced and compare with sequestration capabilities to better understand the net sequestration of salt marshes*
- Better global mapping of the current extent of salt marshes, particularly at high latitudes, as well as habitat-suitability mapping/analysis*
Mechanisms for CDR
- Unlike salt marshes and mangrove forests, seagrass beds remove carbon from seawater, creating a disequilibrium in the CO2 concentration between the seawater and atmosphere. The resulting flux from the atmosphere into the seawater replenishes the deficit in dissolved inorganic carbon due to seagrass photosynthesis. This replenishment may be complete (which maximizes carbon removal) or incomplete (which results in an inefficiency in carbon removal accounting).
- In theory, restoration (e.g., seed/seedling dispersal or planting) is the most likely mechanism for CDR, however, efforts to date are often unsuccessful{{1}}. Restoration propositions are further complicated by the outstanding questions around net carbon removal (especially under different climate change scenarios) and the extent of suitable locations.
- A 2023 study in Australia found that sediment carbon stocks show little recovery immediately after restoration and can take >5 years to return to expected carbon sequestration rates{{2}}.
- Restoration of hydrology, through reversing drainage or removing tidal restrictions, may assist in the recovery and expansion of existing seagrass beds{{3}}. This path may be met with more resistance (especially political and social) than other types of restoration efforts.
CDR Potential
Estimated Sequestration Potential: 0.007 – 0.025 Gt CO2e/year{{4}}
Sequestration Durability: Decades -- 1,000 years{{5}} (Durability of sequestered carbon will depend on multiple variables and can vary greatly between individual ecosystems)
Challenges
- A 2023 review of all available literature on eelgrass (the dominant species of seagrass along the United States west coast) restoration projects in California, Oregon, and Washington found that of the 82 restoration projects, only 6 were published in peer-reviewed journals. This highlights the lack of data availability that can make assessing successes, failures, and best practices difficult{{6}}.
- Difficult to differentiate between autochthonous and allochthonous sources of carbon in sediments{{7}}
- There is substantial inorganic carbon in seagrasses which is derived from outside the seagrass bed{{8}} which in turn has sparked debate over whether seagrasses are ultimately a carbon sink or source.
- Seagrass restoration efforts are often unsuccessful{{9}}
- High variability in carbon burial rates{{10}}
- High cost associated with restoration efforts{{11}}
Key Knowledge Gaps
- Robust measurements of air-water CO2 exchange above seagrass beds
- Global extent of seagrasses (including future extent under changing climate)
- Causes of failed restoration projects
- What are the sources and fates of carbonates in seagrass beds?
First-Order Priorities
*Adapted from the Environmental Defense Fund report on Coastal Natural Climate Solutions{{12}}
- Take additional measurements of air-sea gas exchange above seagrass beds*
- This will lead to the development of more robust predictive models to better understand CDR efficacy.
- Map the global extent of seagrasses and their likely future extent*
- This is likely to involve some combination of active remote sensing and field surveys as well as habitat suitability analyses.
- Mapping of likely future extent will involve considerations of future climate change impacts.
- Continue investigations into the causes of restoration project failures in seagrass systems*
- This effort will likely involve better monitoring, recording, and publication of data on restoration efforts.
- Improve the understanding of the sources and fate of carbonates in seagrass bed sediments*
- This includes the contributions to CO2 production both within seagrass beds and upstream of these ecosystems.
Restoration of coastal vegetated ecosystems can generate many benefits for surrounding marine ecosystems. While we are calling these “co-benefits” here, it should be noted that some of these benefits are so significant (while carbon sequestration feasibility and efficacy remain unknown) that these may in fact be the main benefits of restoration. (Note that there are significantly more co-benefits in coastal vegetated ecosystems than any of the other Ocean Visions’ marine CDR Road Maps.)
- Potential for ocean alkalinity enhancement{{1}}
- Coastal protection against erosion{{2}}
- Nutrient cycling{{3}}
- Pollutant filtering
- Reducing biodiversity loss{{4}}
- Restoring the role of marine organisms in the carbon cycle{{5}}
- Protection against storm surge and inundation{{6}},{{7}},{{8}}
- Providing habitat and nurseries for a variety of marine organisms{{9}}
Restoration of coastal vegetated ecosystems can generate many benefits for surrounding communities and economies. While we are calling these “co-benefits” here, it should be noted that some of these benefits are so significant (while carbon sequestration feasibility and efficacy remain unknown) that these may in fact be the main benefits of restoration. Many of the environmental benefits relate directly to social benefits, such as fisheries enhancement and coastal protection. (Note that there are significantly more co-benefits in coastal vegetated ecosystems than any of the other Ocean Visions’ marine CDR Road Maps)
- Coastal protection from storms, floods, and sea-level rise{{1}}{{2}}{{3}}
- Increased adaptive capacity for communities to handle climate change and natural disasters
- Job creation and economic stimulation from enhanced fisheries and tourism (of particular value to coastal communities that are dependent on marine-based livelihoods)
- Cultural / intrinsic / recreational value
- Education and research – the pursuit of restoring blue carbon for CDR will naturally add value to the existing body of research and can provide valuable educational experiences for the upcoming generation of scientists and conservationists.
Efforts around restoring natural ecosystems are often met with social acceptance and public support, especially when compared to other climate solutions seen as “tampering with nature”{{1}}. This may be in part due to the minimal risks (real and perceived) associated with restoring natural ecosystems. While some risks, outlined below, do exist, there may be a higher tolerance for such risks given the long list of potential benefits and co-benefits (both environmentally and socially).
- Potential for conflict with other industries (aquaculture, fishing, tourism etc.); However, conflicts may be mitigated with participatory approaches to planning and implementation.
- Potential for inequity in benefits: Macreadie et al. (2022) points to concerns around the distribution of benefits from any given blue carbon project and whether benefits are evenly distributed or whether activities reinforce or add to social inequity{{2}}. Similarly, there may be confusion around who “owns” the blue carbon and who has rights to control transactions of credits resulting from blue carbon projects. This may be particularly challenging in marine spaces where the movement of carbon must be considered.
- Develop accessible (economically and geographically) sensors and technologies to measure greenhouse gas exchanges and fluxes both temporally and spatially at high enough resolution to create predictive models for net carbon sequestration and durability.
- Create models that account for future impacts/effects of climate change and land-use change (e.g., coastal squeeze)
- Create new frameworks (e.g., seascape{{1}} or watershed approaches) both for research and financial mechanisms that account for intersystem linkages and take a more holistic approach to measuring carbon associated with coastal vegetated ecosystems.
- Currently, ecosystems are considered and studied individually which can cause issues when thinking about the movement of carbon between systems
- Investigate and characterize the historical or pre-industrial ecosystem locations and ranges (which in many cases are currently unknown) in order to establish baselines for restoration projects.
- In some instances, these prior states may need to be derived from historical sources including more traditional data sets and non-traditional data sets like paintings{{2}}.
Current Landscape
- Verra: In 2020 Verra, a standard for certifying carbon credits, released its first blue carbon conservation methodology, an update to the VCS REDD+ Methodology Framework. Verra has also formed a blue carbon Working Group (2020) that explores opportunities for coastal wetland restoration and conservation as well as developed the Wetland Restoration and Conservation Requirements. Addressing blue carbon in the open ocean, Verra created an Ocean Carbon Working Group as well as the Seascape Carbon Initiative (co-led by Verra, Silvestrum Climate Associates, Blue Marine Foundation, the blue carbon Initiative, and Oceans 2050){{4}}.
- Gold Standard: Gold Standard is a carbon offset standard for non-governmental emission reductions projects. Gold Standard has an approved methodology for the certification of mangrove projects and are exploring other blue carbon opportunities.
- Plan Vivo: Plan Vivo is a certification body that certifies projects against the Plan Vivo Standard, a framework for community and smallholder land-use and forestry projects. In 2014, Plan Vivo certified the world’s first blue carbon project, Mikoko Pamoja (Kenya), for mangrove conservation{{5}}.
- Fair Carbon: Fair Carbon supports the development of blue Carbon projects by increasing the accessibility of information and transparency{{6}}. This includes their Global Blue Carbon Projects map which allows access to all publicly registered blue carbon projects across multiple voluntary carbon market registries. They also have a number of other resources around carbon credits for blue carbon.
- Sustainable Development Mechanism (formerly the Clean Development Mechanism): This is a compliance market designed for shorter-term emissions credits.
- Nationally Determined Contributions (NDCs): These are the climate actions detailed and executed by countries participating in the Paris Agreement and serve as the primary mechanism for meeting the goals set out in the Paris Agreement. The restoration of blue carbon ecosystems may help mitigate and adapt to climate change, however their integration into Nationally Determined Contributions remains underdeveloped{{7}}. A 2022 report from thirty three experts highlights the need for “genuine participation by communities, inclusive project governance, integration of local work into national policies and practices, sustaining livelihoods and income (for example through the voluntary carbon market and/or national Payment for Ecosystem Services and other types of financial compensation systems) and simplification of carbon accounting and verification methodologies to lower barriers to entry{{8}}” in order for blue carbon projects to be responsibly and successfully integrated into Nationally Determined Contributions.
- New efforts to improve the quality of carbon credits and the voluntary carbon market are being discussed and developed{{9}}. The integrity of each carbon credit will be critical to enable the carbon market to address climate change. New guidance has been released in 2023 to ensure carbon credits deliver on their claims. The Integrity Council for the Voluntary Carbon Market released its Core Carbon Principles{{10}} that set a global threshold to define what a high-integrity carbon credit is.
Challenges
- Verification of the amount of carbon sequestered and its durability can be extremely challenging across coastal vegetated ecosystems but is necessary for effective (in terms of mitigating climate change) carbon markets.
- Ensuring fairness and equity in benefits distribution
Seaweeds use photosynthesis to convert dissolved carbon in the surrounding seawater into organic compounds and living tissue{{1}}. As dissolved carbon is removed from the ocean, it is replaced by carbon dioxide from the atmosphere. It is through this process that seaweed can remove CO2 from the atmosphere. The ultimate fate of carbon sequestered in living seaweed is varied and includes becoming remineralized, or converted back, to CO2 via grazing, exuded as dissolved organic carbon, and being transported to the deep sea as particulate matter. The rate and proportion of carbon through each of these pathways remains uncertain{{2}}, though a 2016 study from Krause-Jensen and Duarte sheds some light on possible export efficiencies{{3}}. What’s more, the rate and proportion of carbon that goes through each of the pathways mentioned are not only uncertain but context-dependent. For an in-depth exploration of the readiness of seaweed as a blue carbon solution (both natural stands and farmed) see Fujita et al. 2023{{4}}.
Note that this road map will exclusively look at the ability of natural seaweed stands and coastal seaweed farms to passively sequester CO2 (without active human intervention). For an in-depth look at growing seaweed at large scale to be sequestered using active human intervention (such as deep sea sinking and production of algal biochar), please see the Macroalgae Cultivation and Carbon Sequestration Road Map.
[post_title] => Overview [post_excerpt] => [post_status] => publish [comment_status] => closed [ping_status] => closed [post_password] => [post_name] => overview [to_ping] => [pinged] => [post_modified] => 2023-12-01 18:20:29 [post_modified_gmt] => 2023-12-01 18:20:29 [post_content_filtered] => [post_parent] => 4034 [guid] => http://ocean-visions.local/roadmaps/living-blue-carbon/introduction-to-the-roadmap/overview-6/ [menu_order] => 0 [post_type] => page [post_mime_type] => [comment_count] => 0 [filter] => raw )Here, natural seaweed stands denotes seaweed naturally occurring in the ocean, also commonly referred to as kelp forests.
Mechanisms for CDR
- Reforestation of natural seaweed stands is the process of assisting in the recovery of seaweed stands that have been degraded, damaged, or destroyed, and is a type of restoration.
- The reforestation of natural seaweed stands to increase carbon storage involves many context-specific factors that may complicate such efforts. Factors that will affect the growth and ultimate success of seaweed include climate change, disease, grazers, and predator interactions, etc.{{1}}. It is also highly species dependent with some species of seaweed better suited than others for CDR. This complex assemblage of factors, unique across geographies, will require restoration efforts to be highly tailored.
- A 2022 meta-analysis of seaweed restoration projects found a high success rate of restoration projects, however many of the reported projects were small in scale and these findings may not reflect failed restoration projects that were not reported{{2}}.
- The Nature Conservancy’s guidance on developing kelp restoration projects{{3}}
- One emerging method for restoring degraded seaweed stands is seeding small rocks with juvenile kelp, often termed ‘Green Gravel’. See work by the Green Gravel Action Group.
- Another proposed technique actively removes urchins from seaweed stands to allow for the settlement of juvenile seaweed and subsequent regrowth. See Urchinomics for one approach.
- Increasing productivity of existing seaweed stands via genetic manipulation
- There is current research on improving strain selection for enhanced carbon sequestration, productivity, or resiliency of seaweed. See ongoing research by Charles Yarish and lab at the University of Connecticut.
CDR Potential
Estimated Sequestration Potential: 0 - 0.018 Gt CO2e /year{{4}}
Sequestration Durability: Potentially hundreds of years, however, more studies are needed to understand how much seaweed becomes “refractory”, or resistant to degradation on the timescale of hundreds of years{{5}}.
Environmental Co-Benefits
Restoration of natural seaweed stands may generate many benefits for surrounding marine ecosystems. While we are calling these “co-benefits” here, it should be noted that some of these benefits may be so significant that these may in fact be the main benefits of restoration. (Note that there are significantly more co-benefits in blue carbon than any of the other marine CDR Road Maps.)
- Potential amelioration of ocean acidification{{6}}
- Contribution to biodiversity{{7}}
- Contribution to habitat provisioning{{8}}
- Contribution to heavy metal and nutrient pollution removal{{9}},{{10}}
- Contribution to fishery enhancement{{11}},{{12}}
- Improved water clarity through the facilitation of settlement of fine sediments{{13}}
- Nutrient cycling{{14}}
- Reducing biodiversity loss{{15}}
- Restoring the role of marine organisms in the carbon cycle{{16}}
Environmental Risks
In contrast to other proposed marine CDR pathways, there are not as many apparent environmental risks to reforesting natural seaweed stands for carbon dioxide removal. However, proper restoration techniques which take context-specific factors into consideration are critical to ensuring no or limited negative environmental impacts. As with any CDR pathway, incremental scale-up with careful monitoring and verification of impacts will be critical to mitigate risks. Possible environmental risks may include:
- Species entanglement
- Nutrient competition
- Smothering of adjacent sensitive ecosystems
- Negative effects of organic exudates on neighboring ecosystems
- If hard bottom substrates are needed (for seaweed to attach to) this could disturb soft bottom habitats. See examples of artificial reef creation to mitigate the loss of natural reef and restore seaweed.
Social Co-Benefits
Reforestation of natural seaweed stands may generate benefits for surrounding communities and economies. While we are calling these “co-benefits” here, it should be noted that some of these benefits may be so significant (while carbon sequestration feasibility and efficacy remain unknown) that these may in fact be the main benefits of restoration. Many of the environmental benefits relate directly to social benefits, such as fisheries enhancement and coastal protection. (Note that there are significantly more co-benefits in blue carbon than any of the other CDR Road Maps.)
- Increased adaptive capacity for communities to handle climate change and natural disasters via mechanisms such as wave attenuation for coastal protection{{17}}, providing a refugia from acidified waters that benefits shellfish{{18}}, mitigation of dead zones by production of dissolved oxygen{{19}}, or possibly providing shading from seaweed blades to create a thermal refugia during marine heat waves{{20}}
- Job creation and economic stimulation from enhanced fisheries and tourism (of particular value to coastal communities that are dependent on marine-based livelihoods)
- Cultural / intrinsic / recreational value
- Education and research – the pursuit of restoring blue carbon for CDR will naturally add value to the existing body of research and can provide valuable educational experiences for the upcoming generation of scientists and conservationists.
Social Risks
Efforts around restoring natural ecosystems are often met with social acceptance and public support, especially when compared to other climate solutions seen as “tampering with nature”{{21}}. This may be in part due to the minimal risks (real and perceived) associated with restoring natural ecosystems. While some risks, outlined below, do exist, there may be a higher tolerance for such risks given the long list of potential benefits and co-benefits (both environmentally and socially).
- Potential for inequity in benefits: Macreadie et al. (2022) points to concerns around the distribution of benefits from any given blue carbon project and whether benefits are evenly distributed or whether activities reinforce or add to social inequity{{22}}. Similarly, there may be confusion around who “owns” the blue carbon and who has rights to control transactions of credits resulting from blue carbon projects. This may be particularly challenging in marine spaces where the movement of carbon must be taken into account.
- Potential for conflict with other uses such as commercial fisheries, shipping, marine renewable energy, and mining{{23}} however, there may be synergies for some industries, like utilizing infrastructure from nearby renewable energy for monitoring.
Key Knowledge Gaps
Adapted from EDF 2022 Carbon Sequestered by Seaweed{{24}}
- What is the best way to measure rates of carbon sequestration by natural seaweed stands?
- Which of the carbon fluxes between natural seaweed stands and their environment are well quantified, and which are not?
- How does carbon sequestered by natural seaweed stands compare with other ocean-based carbon sequestration pathways?
- Is the restoration of natural seaweed stands a durable and feasible enough pathway to help stabilize the climate?
*Note that all of these are highly dependent upon what species of seaweed is being considered.
First-Order Priorities
*Adapted from EDF 2022 Carbon Sequestered by Seaweed{{24}}
- Conduct research to better understand and characterize carbon fluxes in natural seaweed stands
- Determine the best way to measure carbon sequestration rates by natural seaweed stands*
- Determine which of the carbon fluxes relevant to carbon sequestration by seaweeds are well quantified and which are not. This includes carbon fluxes from the atmosphere to ocean, ocean to seaweed, seaweed to microbial and other food webs, and seaweed to deep water*.
- Characterize how the potential carbon sequestration by natural seaweed stands compares with other ocean-based carbon sequestration pathways*
- Determine the durability of carbon sequestered via natural seaweed stands and if it is long enough to help stabilize the climate*
- Accelerate the innovation and testing of new technology that can aid in restoration projects
- Conduct further field testing of techniques such as green gravel for afforestation of kelp forests.
- Conduct more research on the efficacy of utilizing genetic manipulation and biotechnology to enhance seaweed carbon capture efficiencies.
Mechanism for CDR
Expansion of seaweed farming
- Possibly the most promising pathway to increase carbon sequestration by seaweed due to rapid advances in infrastructure, farm operations, and monitoring.
- Achieving additional, passive, carbon sequestration would require biomass to be left in the ocean long enough for fragmentation, transport, and burial to occur.
CDR Potential
Estimated Sequestration Potential: 0.00144 – 0.0079 Gt CO2e/year{{1}}
Sequestration Durability: Potentially hundreds of years, however, more studies are needed to understand how much seaweed becomes “refractory”, or resistant to degradation on the timescale of hundreds of years{{2}}.
Environmental Co-Benefits
The creation or expansion of seaweed farms may generate benefits for surrounding marine ecosystems, including but not limited to:
- Potential amelioration of ocean acidification{{3}}
- Contribution to biodiversity{{4}}
- Contribution to habitat provisioning{{5}}
- Contribution to heavy metal and nutrient pollution removal{{6}},{{7}}
- Contribution to fishery enhancement{{8}},{{9}}
- Improved water clarity through the facilitation of settlement of fine sediments{{10}}
- Nutrient cycling{{11}}
- Restoring the role of marine organisms in the carbon cycle{{12}}
Environmental Risks
- Burning of fossil fuels associated with production activities (harvest, transport, processing)
- Production of CH4, N2O, and other potentially hazardous gases by the macroalgae
- Impacts to biodiversity and ecosystem function from farming operations may include: enhanced disease and parasite risk, alteration of population genetics, introduction of non-native species into new environments
- Enhancement in epiphytic calcifiers that could offset carbon sequestration through calcification-induced CO2 release.
- Reduced phytoplankton production in and around seaweed farms due to competition for nutrients and light
- Changes in light and nutrient availability (including possible changes in ocean albedo due to canopy cover)
- Entanglement of marine megafauna
- Addition of noise pollution due to vessel traffic and machinery
Social Co-Benefits
Seaweed farming may generate many benefits for surrounding communities and economies.
- Basis for circular marine bio-economies, generating multiple benefits{{13}} including integrated multi-trophic farming efforts to collocate multiple species (i.e., seaweed and shellfish)
- Education and research – the pursuit of restoring blue carbon for CDR will naturally add value to the existing body of research and can provide valuable educational experiences for the upcoming generation of scientists and conservationists.
Social Risks
While some risks, outlined below, do exist, there may be a higher tolerance for such risks given the long list of potential benefits and co-benefits (both environmentally and socially).
- Potential for conflict with other industries (fishing, tourism etc.){{14}}
- Potential for conflict with other uses such as commercial fisheries, shipping, marine renewable energy, and mining{{15}} (However, note there are also possible synergies that may exist such as co-location of seaweed farms with compatible marine infrastructure types such as the moorings for wind energy, which could reduce costs for both industries.)
Challenges
- Scaling
- Rough seas, light and nutrient availability, and the availability of space are all constraints.
- Measuring Carbon
- Difficulties in measuring and verifying how much carbon is being sequestered passively by the presence of seaweed farms.
Key Knowledge Gaps
*Adapted from EDF 2022 Carbon Sequestered by Seaweed{{16}}
- What is the best way to measure rates of carbon sequestration by seaweed farms?
- Which of the carbon fluxes between seaweed farms and their environment are well quantified and which are not?
- How does carbon sequestered by seaweed farms compare with other ocean-based carbon sequestration pathways?
First-Order Priorities
*Adapted from EDF 2022 Carbon Sequestered by Seaweed{{16}}
- Investigate and characterize carbon fluxes in coastal seaweed farms
- Determine the best ways to measure rates of carbon sequestered by seaweed farms*
- Investigate which carbon flows relevant to quantifying carbon sequestration by seaweed farms are well documented and which remain uncertain*
- Estimate total greenhouse gas emissions (including N2O and CH4) and carbon sequestration associated with seaweed farms{{17}}*
Whales are thought to play a role in the carbon cycle both directly (living biomass and whale falls) and indirectly (the whale pump, the whale conveyor belt, and bioturbation), however the extent of this role remains uncertain{{1}}. A 2022 report from The Environmental Defense Fund{{2}} found there to be fewer than 10 scientific studies from which to draw any broad conclusions in this area and cautioned against the creation of a carbon credit system based on whale carbon sequestration. A 2020 study estimates that restoring baleen whale populations to pre-whaling abundances could sequester 0.032 Gt CO2/year, including carbon sequestered from fertilization via whale feces{{3}}. However, rebuilding whale populations is sure to elicit many other environmental and social benefits, explored further on in this road map.
Mechanisms for CDR
Direct Sequestration
- Living Biomass: Due to their size and longevity, whales can store large amounts of carbon for long periods of time in their bodies as biomass. While whaling indeed resulted in a loss of carbon stored in whales, there is not clear evidence to suggest that whaling was a net source of CO2 to the atmosphere{{4}}.
- Whale Falls: Whale falls, wherein whale carcasses sink to the ocean floor and their carbon-rich biomass is reallocated in various ways, is the “most scientifically comprehensible” of the pathways (direct vs indirect sequestration){{5}} with a 2010 study estimating that restoration of large baleen whale populations to pre-whaling numbers could sequester 0.0006 Gt CO2e /year.
Indirect Sequestration
- Whale Pump: This is the term often used to describe the role that whales play in cycling nutrients through the ocean via their feces. The whale pump hypothesis sits on two assumptions that have not yet been verified through empirical observations{{6}}. The first assumption is around the efficacy of surface water fertilization and subsequent primary production via whale feces. The second assumption concerns the fate of carbon export from primary production.
- WhaleX Ocean Nourishment synthesizes limiting nutrients (nitrogen, phosphorous, iron, and silica) and disperses them across nutrient poor regions of the ocean, simulating the dispersion of nutrients via whale feces.
- Whale Conveyor Belt: The whale conveyor belt is based on the idea that as whales migrate yearly, they move nutrients around the ocean, potentially increasing iron availability for phytoplankton growth{{7}}.
- Bioturbation: Bioturbation is the process through which whale feeding behaviors stimulate the seabed and resuspend sediments{{8}}. Conceptually, this is similar to the whale pump, but the pool of nutrients comes from seafloor sediments instead of whale feces{{9}},{{10}}.
Rebuilding Whale Populations: Increasing whale populations would likely amplify their role in the carbon cycle, including any sequestration.
- Increasing Krill Abundance:
- A 2022 article by Savoca{{11}} suggests krill as a limiting factor to whale population growth. Savoca points to studies that suggest a close relationship between whales and krill wherein whales provide necessary nutrients for krill to thrive. This is further complicated by competition between krill fisheries and whales in the Southern Ocean.
Reducing Ship Strike: A 2001 study found that in some areas, more than one third of all fin whale and right whale strandings involve ship strike. This can especially impact small populations of whales such as the northern right whale in the western North Atlantic{{12}}. Measures that require large, motorized vessels to slow their speeds in particularly vulnerable areas may be beneficial to whale populations.
CDR Potential
Estimated Sequestration Potential: 0.032 Gt CO2e /year, including carbon sequestered from fertilization{{13}}
Sequestration Durability: 10 – 100 years{{14}}
Challenges
- Difficult to find and track outcomes from whale fertilization events (e.g., feces)
- Tracking the fate of carbon through the water column
Key Knowledge Gaps
Adapted from Pearson et al. 2022
- How effective are whales at creating and maintaining primary production hotspots that lead to enhanced carbon sequestration?
- How bioavailable are whale-derived nutrients?
- What is the carbon flux from cetaceans to the atmosphere?
First-Order Priorities
*Adapted from Pearson et al. 2022
- Investigate primary production hot spots from whales via determination of fecal nutrients to carbon recycling versus export*
- Conduct additional field and laboratory studies to ascertain the bioavailability of krill-derived iron in whale feces*
- Measure the carbon flux from cetaceans to the atmosphere via respiration to understand cetaceans’ net capacity for CO2 removal*
While fishes have not traditionally been considered as important parts of the ocean carbon cycle / biological pump{{1}}, there are several recent studies, as highlighted in the 2022 Environmental Defense Fund report “Natural Climate Solutions in the Open Ocean”, that focus on the geochemical contributions of fishes to the global carbon cycle{{2}}{{3}}{{4}}{{5}}{{6}}. Many of the mechanisms by which fish are thought to contribute to carbon cycling are similar to whales (carbon stored in living biomass, bioturbation, carcasses falling to ocean floor, fertilization through feces) but also include contribution by intestinal precipitation of calcium carbonates{{7}}.
There is still uncertainty around the specifics of mesopelagic fish-mediated carbon export, however the 2022 Environmental Defense Fund report suggests there is enough evidence of these species’ large contributions to global sequestration to warrant “the immediate pursuit of limitations or prohibitions on their harvest”.
Mechanism for CDR
Limitations or prohibitions on harvest may increase the living biomass of fish, inducing higher natural long-term carbon sequestration by increasing carcass deadfall. Previous studies that evaluate the effectiveness of reducing fishing and establishing marine protected areas to address climate change suggest that these efforts support climate adaptation{{8}}.CDR Potential
Estimated Sequestration Potential: Unclear, due to large knowledge gaps around biomass estimates of marine fish stocks, uncertainties in direct and indirect carbon export and overall carbon fluxes, few studies observing natural senescence / dead falls of fishes, and a poor understanding of the life cycle emissions of most fish species{{9}}.
Sequestration Durability: Unknown.
Challenges
- More research is needed to understand carbon fluxes and life cycle emissions before developing strategies to increase carbon uptake and sequestration.
Key Knowledge Gaps
Adapted from EDF Natural Climate Solutions in the Open Ocean
- Lack of knowledge around the natural senescence or resulting deadfalls of fishes
- What is the contribution of fishes to inorganic carbon export via carbonate excretion?
- What is the total biomass of fishes, the fluxes in these communities, and conversion factors to go from biomass to units of carbon?
- How to discern between active carbon transport from mesopelagic fish versus from zooplankton?
- What is the composition of mesopelagic communities and what are the differences in roles played by small fishes versus other species such as cephalopods?
First-Order Priorities
*Adapted from EDF Natural Climate Solutions in the Open Ocean
- Conduct studies observing the natural senescence or resulting deadfalls of fishes*
- Conduct additional studies to understand the contribution of fishes to inorganic carbon export via carbonate excretion{{10}}*
- Create new models, observing technologies, and data to better constrain the total biomass of fishes, the fluxes in these communities, and conversion factors to go from biomass to units of carbon*
- Create new observational methods and models to better discern between active carbon transport from mesopelagic fish versus from zooplankton*
- Conduct studies to better understand the composition of mesopelagic communities to determine the difference in roles played by small fishes versus other species such as cephalopods*
- Nutrient cycling{{1}}
- Restoring the role of marine organisms in the carbon cycle{{2}}
Restoration of marine animal populations may generate many benefits for surrounding communities and economies. While we are calling these “co-benefits” here, it should be noted that some of these benefits may be so significant (while carbon sequestration feasibility and efficacy remain unknown) that these may in fact be the main benefits of restoration. Many of the environmental benefits relate directly to social benefits, such as fisheries enhancement and coastal protection. (Note that there are significantly more co-benefits in “blue carbon” than any of the other CDR Road Maps.)
- Potential for economic stimulation and job creation from enhanced tourism (e.g., whale watching - of particular value to coastal communities that are dependent on marine-based livelihoods)
- Cultural / intrinsic / recreational value
- Education and research – the pursuit of restoring blue carbon for CDR will naturally add value to the existing body of research and can provide valuable educational experiences for the upcoming generation of scientists and conservationists.
Efforts around restoration efforts are often met with social acceptance and public support, especially when compared to other climate solutions seen as “tampering with nature”{{1}}. This may be in part due to the minimal risks (real and perceived) associated with restoration efforts. While some risks, outlined below, do exist, there may be a higher tolerance for such risks given the long list of potential benefits and co-benefits (both environmentally and socially).
- Potential for negative economic impacts to some industries such as oil and gas, fisheries, and mining{{2}}
- Stricter fisheries management to increase species abundances can cause impacts on food security, displacement, and economic strain. (for example, Marine Protected Areas can cause varied impacts among communities and social groups and can be met with opposition){{3}}
Some restoration efforts (particularly those most studied such as mangroves and salt marshes) are generally feasible, local, and have high local governability{{1}}, however the scale and durability of these efforts will be critical to their success as effective CDR pathways. The restoration of marine habitats and species aligns with several broad-reaching international agreements and domestic laws{{2}}. Several international agreements and policies that recognize the value and importance of restoring marine life and ecosystems and that may be important when considering scaling up restoration efforts include:
- Parties to the United Nations Convention on the Law of the Sea (UNCLOS)
- The Convention on Biological Diversity (CBD)
- The United Nations Decade of Ecosystem Restoration (2021-2030)
- UNFCCC Paris Agreement
- Coastal ecosystems can be included in Nationally Determined Contributions (NDCs)
- Global Biodiversity Framework
- Commission for the Conservation of Antarctic Marine Living Resources (CCAMLR)
- UN High Seas Treaty (BBNJ Treaty)
- The Habitats Directive{{3}} (European Commission)
Examples of agreements aimed at protecting marine life
- The Endangered Species Act (United States)
- The Marine Mammal Protection Act (United States)
- The National Marine Sanctuaries Act (United States)
- Marine Protected Areas (Note that MPAs are designed to protect and conserve biodiversity, rather than carbon. For coastal systems, these will be located in national or state waters.)
Examples of US Blue Carbon Policy
- Executive Order 14008: Tackling the Climate Crist at Home and Abroad (2021): Places the climate crisis at the forefront of foreign policy and national security planning.
- America the Beautiful Initiative: Outlines inclusive and collaborative plan for locally led efforts to conserve, steward, and restore land and waters.
- Justice 40 Initiative: A national commitment to environmental justice which includes investments in the reduction of legacy pollution.
- Ocean Climate Action Plan (OCAP): Includes ongoing and planned federal ocean-based climate mitigation and adaptation activities and recommends new or enhances ocean science and policy actions to tackle climate change. It also includes environmental justice considerations into ocean-based climate solutions.
- CREST Act of 2023: An expansion on the current research and development programs of the Department of Energy for capturing and storing carbon dioxide to include methods like CDR.
- Coastal Habitat Conservation Act of 2023: Authorizes the appropriation of $111.5 million over the 2024-2028 period for the US Fish and Wildlife Service to implement the Coastal Program.
- Prioritize leadership and partnership with local communities and recognize formal and informal tenure agreements.
- Networks of nations (e.g., International Partnership for Blue Carbon) can aid in building capacity to implement policies that support blue carbon projects.
- New IPCC inventory guidance is needed on emissions inventories for climate mitigation resulting from restoring seagrass, seaweed, and other blue carbon ecosystems (the current lack of guidance here also limits private sector investment)
- Property rights, coastal and offshore, are often unclear, not well defined, or not enforced, begging the question, who has jurisdiction over what. This is further complicated by the lateral movement of carbon in blue carbon ecosystems.
- Local governance of global commons is further complicated by local contexts, management structures, and benefit-sharing rules{{1}}.
- Permitting may also pose an additional obstacle for some restoration projects.
- Regulations for coastal developments could impede coastal vegetated ecosystem restoration and limit the ability to launch larger restoration projects.
- Pacific Northwest Blue Carbon Working Group
- Coastal Carbon Research Coordination Network
- International Partnership for Blue Carbon
- The Blue Carbon Initiative
- The Blue Carbon National Working Group
- Blue Carbon for Our Planet Act
- The Blue Boat Initiative
- Blue Carbon Network (Pew)
- Blue Carbon Lab (Deakin University)
- Blue Carbon Canada (UN Decade Action)
- Global Mangrove Watch
- Blue Carbon Calculator
- Blue Carbon Explorer (TNC) (Map)
- Coastal Carbon Atlas
- Blue Carbon Action Partnership
- Global Ocean Decade Programme for Blue Carbon (UN Decade Program)
- Blue Ventures (GEM etc)
- Blue Carbon Accelerator Fund
- Sea4soCiety
- Delta Blue Carbon (Pakistan)
- Global Mangrove Alliance
- Mangrove Action Project (US)
- Blue Carbon Lab (Australia)
- Vida Manglar Carbon Project (Colombia)
- Ocean Alive (Portugal)
- MedGardens (Mallorca)
- ResilienSEA (West Africa)
- Ozfish (Australia)
- Project Seagrass (UK & Globally)
- For an example of a successful seagrass bed restoration, see Orth et al. 2020{{1}}.
Blue Carbon
Restoring blue carbon systems for CDR